IQS Research
Introducing our research fields at IQS Berlin
Atomic Vapor-Cell based Optical Frequency References
Timekeeping for next generation global navigation satellite systems
Optical frequency references have long surpassed the stability of their microwave counterparts in lab environments. Remaining challenge to make use of these devices in field experiments is a more compact and robust design at the same performance level. Our aim is to develop microintegrated optical references comprising atomic vapor cells towards low size, weight and power (SWaP) atomic clocks. We focus on systems utilizing transitions beyond the D2 line in rubidium, such as two-photon 5S → 5D, to reach stabilities regimes relevant for global navigation satellite systems (GNSS).
Team: J. Kluge, D. Kohl, M. Eisebitt, M. Müller, J. Wollenberg, Dr. K. Döringshoff
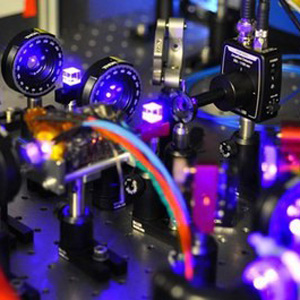
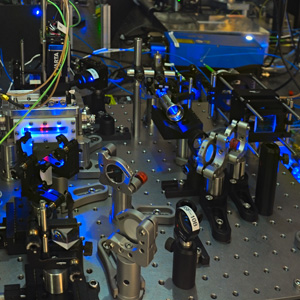
Strontium Optical Clocks
Clock technologies based on thermal and ultracold strontium for space and industry
One natural atomic candidate for precision clock applications is strontium employing a rich electronic term structure featuring broad, narrow and ultra-narrow transitions. In one type of clocks currently developed in our group, we utilize Ramsey-Bordé Interferometry on a thermal strontium beam. By developing compact physics packages including, e.g., low SWaP ovens, these activities aim for an atomic beam clock fitting inside a 19“ rack, suitable for operation in space with fractional frequency stabilities of below 10-15. Other activities use 88Sr atoms, which will be laser-cooled, trapped and interrogated in a 1D optical lattice. Maturing these technologies for space applications opens up new possibilities for fundamental science like clock based gravitational wave detection, searches for dark matter and tests of local position invariance.
Team: O. Fartmann, L. Wihan, C. Pyrlik, Dr. A. Mahdian, Dr. H. Zimmermann, Dr. Ingmari Tietje, Dr. V. Schkolnik
Miniaturization of Atomic Physics Packages
Enabling technologies for UHV compatible integration and additive manufacturing
Miniaturization of experimental setups and optical systems is the key towards the next generation of compact quantum sensor technology and is opening up new prospects in numerous fields of application. Our activities include modeling of optical components, hybrid micro-integration of electro-opto-mechanical devices, system design and verification. In particular we investigate the use of additive manufacturing of ceramics and thermoplastics for compact and scalable assemblies, the development of miniaturized free-space optical systems for atomic manipulation in UHV environments and the qualification of joining techniques for use in UHV systems and space applications.
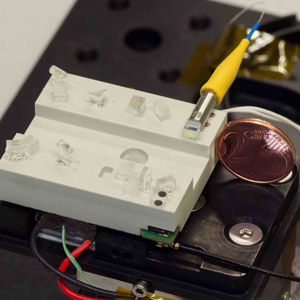
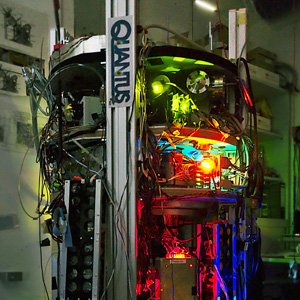
Atom Optics and Interferometry with Bose-Einstein Condensates
Engineering of coherent matter wave packets in free fall
Quantum sensors based on ultracold atoms are promising candidates to impact our future daily lives with applications in navigation systems and geodesy, but are also proposed for tests of fundamental physics with unrivaled sensitivities. Due to the point source-like characteristics and low expansion velocities, BECs constitute ideal quantum probes for matter-wave interferometry. In absence of gravity, the free and unperturbed evolution of a BEC can be extended significantly, which is crucial for entering new regimes in high-precision measurements. Our part in the QUANTUS collaboration comprises ground work with conceptual studies including the preparation of matter-wave packets as source for novel interferometric schemes and BEC interferometry in microgravity towards testing the universality of free fall with quantum matter.
Team: S. Kanthak, Dr. J. Pahl
Laser System Development for Harsh Environments
Diode laser systems for cold atomic devices on mobile platforms
We focus on development of compact and robust high power laser systems, which are key technology for the generation and coherent manipulation of cold atomic gases. Each mobile platform features its own challenging environment. We work on ground-based microgravity platforms, such as the ZARM drop tower in Bremen, and in future also on the Einstein Elevator in Hannover. Each platform sets high demand on the size, weight and power consumption of the experiment, and especially the laser systems must withstand high accelerations during operation and maintain frequency and intensity locks. Even higher demands are imposed for laser systems on space-borne microgravity platforms, like sounding rockets or small satellites. Here, we are interested in hard- and software tools for stand-alone systems with reliable autonomous operation.
Team: O. Anton, S. Kanthak, A. Ukhanova, Dr. J. Pahl, Dr. I. Papadakis, Dr. V. Schkolnik
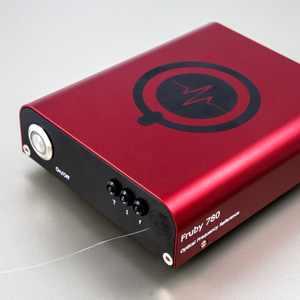
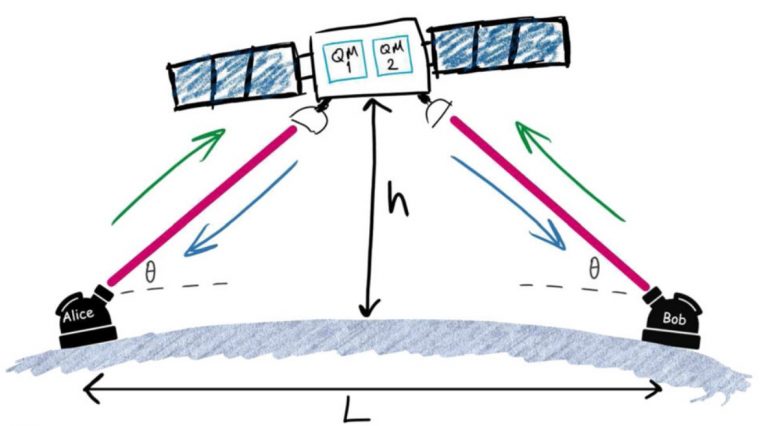
Atomic Quantum Memories in Space
Towards physically secure, long-distance communications
Quantum communication is usually limited to around a few hundred kilometers due to the exponential losses in optical fibers. Quantum repeaters (QR) based on heralded storage of entangled states have been proposed to overcome this direct transmission limit. However, they are still limited to around a few thousand kms. On the other hand, space-based quantum links where channel loss scales mainly polynomially offer another solution to this problem. In this case, however, the communication distance is limited to the line-of-sight distance of the satellite which is around 2000 km for low earth orbit. Within this context, in order to reach truly global distances, we are working on QR architectures operating in space, including experimental work towards building space-compatible quantum memories with warm and cold atomic gases.
Team: M. Jutisz, Y. Murat, M. Schlösinger, Dr. E. Da Ros, Dr. M. Gündoğan
Atomic ensemble based sensors
Integrated devices for magnetomyography and velocimetry
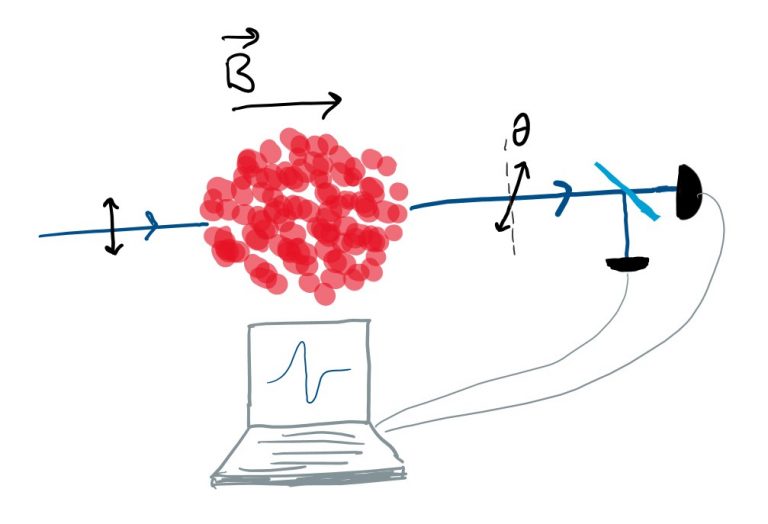
Funded and/or supported by:
